Importance of Target Engagement in Drug Discovery
Despite billions invested in drug development, more than 90% of clinical drug candidates fail. A lack of target engagement causes a significant proportion of these failures—the drug’s inability to interact with its intended biological target to achieve the desired effect1. When drugs fail to engage their targets adequately, they often lack efficacy, leading to clinical failure. The importance of target engagement is underscored by the fact that nearly 50% of new drug candidates fail due to inadequate efficacy, often linked to poor target engagement1. This blog explores the reasons behind target engagement failures and discusses emerging solutions to improve drug development outcomes.
Reasons for Target Engagement Failures
1. Inadequate Preclinical Target Validation
One of the primary reasons for target engagement failures is inadequate preclinical target validation. Preclinical studies are essential for establishing the biological relevance of a target and confirming that modulation of the target leads to the desired therapeutic effect. However, many preclinical models are unreliable, and the results may not translate to humans. For instance, in oncology, many preclinical models fail to predict clinical efficacy accurately, leading to Phase III failures 2.
2. Poor Target Engagement in Clinical Trials
Poor target engagement can lead to failure even when a drug candidate progresses to clinical trials. This can occur due to several factors, including:
- Insufficient Drug Concentrations at the Target Site: If the drug does not reach sufficient concentrations at the target site, it may fail to engage the target effectively. This can be due to poor pharmacokinetics or inadequate dosing regimens3.
- Low Binding Affinity or Selectivity: Drugs with low binding affinity or poor selectivity may fail to effectively engage the target, leading to a lack of efficacy or off-target effects4.
- Complexity of Target Biology: Some targets are inherently complex, with multiple isoforms, post-translational modifications, or dynamic protein-protein interactions. These factors can make it challenging to achieve effective target engagement5.
3. Lack of Effective Biomarkers and Measurement Tools
Another significant challenge is the absence of robust biomarkers and tools to measure target engagement in clinical trials. Biomarkers are essential for confirming that a drug engages its target and induces the desired pharmacodynamic response. Without such tools, assessing whether the lack of efficacy is due to poor target engagement or other factors is challenging. Advanced techniques, such as CETSA® technology, are valuable for assessing target engagement in a physiological setting.
4. Inadequate Understanding of Target Biology
A poor understanding of the target’s biology can also contribute to target engagement failures. For example, in Alzheimer’s disease, the complexity of the underlying biology and the dynamic nature of amyloid and tau pathology have made it challenging to develop drugs that engage their targets effectively6. Gaining deeper biological insights and leveraging advanced methodologies can help address these challenges.
Consequences of Target Engagement Failures
Target engagement failures result in costly clinical trial failures, delays in bringing new therapies to patients, and a substantial financial burden on the pharmaceutical industry. Moreover, failed drugs can also harm patients by exposing them to ineffective or potentially harmful treatments2.
Strategies to Improve Target Engagement with CETSA
Understanding why drugs fail due to poor target engagement is only part of the challenge. Equally important is identifying strategies to mitigate these risks. Below are some approaches that leverage CETSA® technology to improve drug development outcomes:
1. CETSA for Quantifying Target Engagement in Physiological Conditions
CETSA (Cellular Thermal Shift Assay) enables researchers to measure target engagement directly in intact cells and tissues, preserving physiological conditions. Unlike traditional methods requiring chemical modifications or engineered labels, CETSA provides a label-free, unbiased assessment of drug-target interactions, making it a valuable tool for preclinical and clinical research.
2. Early Identification of Compounds with Poor Target Engagement
By integrating CETSA in early-stage screening, researchers can rapidly assess whether a compound effectively binds its target. This enables the elimination of weak candidates before costly clinical trials, reducing failure rates and improving efficiency in drug development.
3. Enhancing Biomarker Development
CETSA facilitates the discovery of reliable biomarkers linked to target engagement, helping researchers assess pharmacodynamic responses in real-world biological settings. This supports better dose selection, treatment optimization, and personalized medicine strategies.
4. Enhanced Preclinical Target Validation
Improving preclinical target validation is critical for reducing clinical attrition. Using CETSA as part of preclinical validation enhances confidence that a drug engages its target under relevant physiological conditions. This strengthens the translation of preclinical findings into clinical success by minimizing false positives that arise from artificial assay conditions.
Conclusion
Target engagement failures remain a leading cause of drug development setbacks. However, technologies like CETSA are transforming how researchers assess drug-target interactions, offering label-free, physiologically relevant insights. By integrating CETSA early in the development process, pharmaceutical companies can improve success rates, reduce costly late-stage failures, and bring more effective therapies to patients.
To learn more about real-world applications of CETSA and explore case studies, visit our Scientific Hub today.
References
- Sun et al. Acta Pharm Sin B. 2022
- Seruga et al. Clinical Cancer Research. 2015
- Durham et al. AAPS Advances in the Pharmaceutical Sciences Series. 2017
- Stefaniak et al. ACS Med Chem Lett. 2020
- Gholizadeh et al. Middle East Journal of Rehabilitation and Health Studies 2021
- Yaari et al. Clin Invest. 2015
Download valuable CETSA® resources here
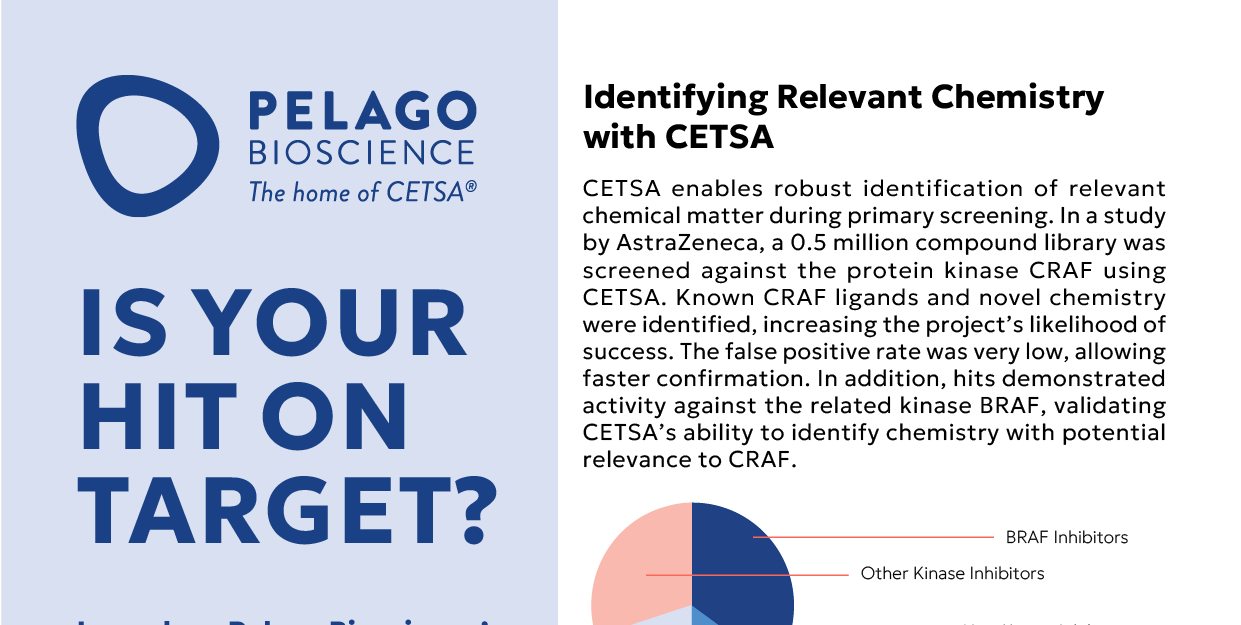
Is Your Hit on Target?
Validate Early with CETSA® Accelerate hit confirmation and lead optimization with CETSA® …
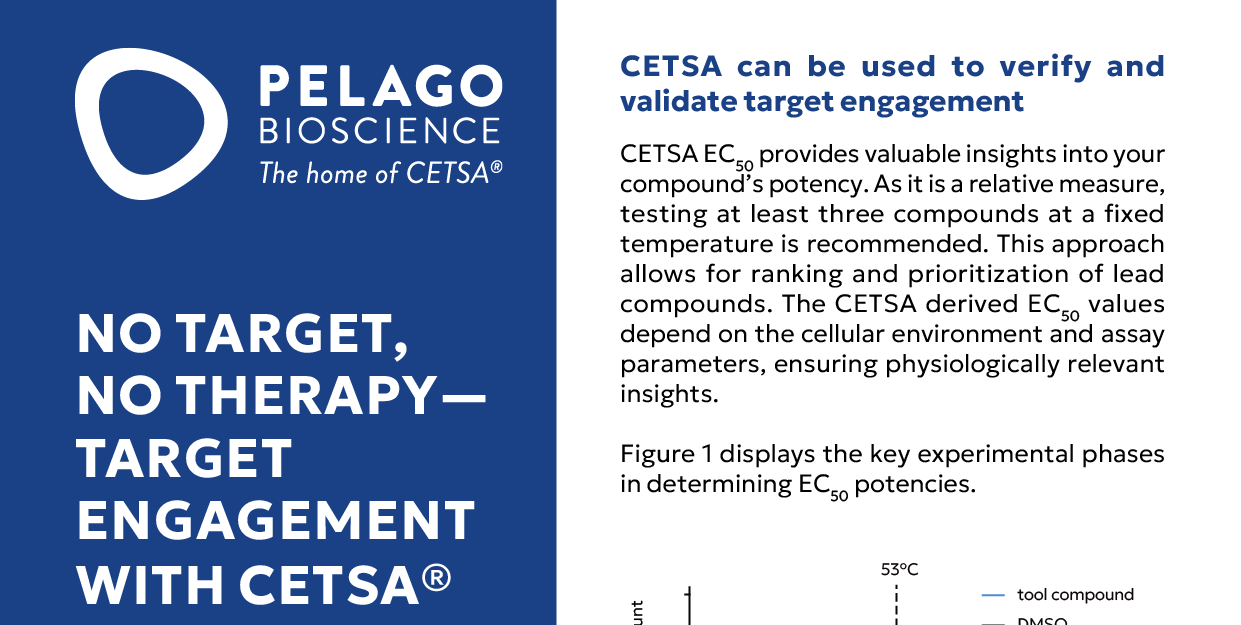
No Target, No Therapy – Target Engagement with CETSA®
Direct Insights into Target Engagement with CETSA® Discover how potency ranking with CETSA help…
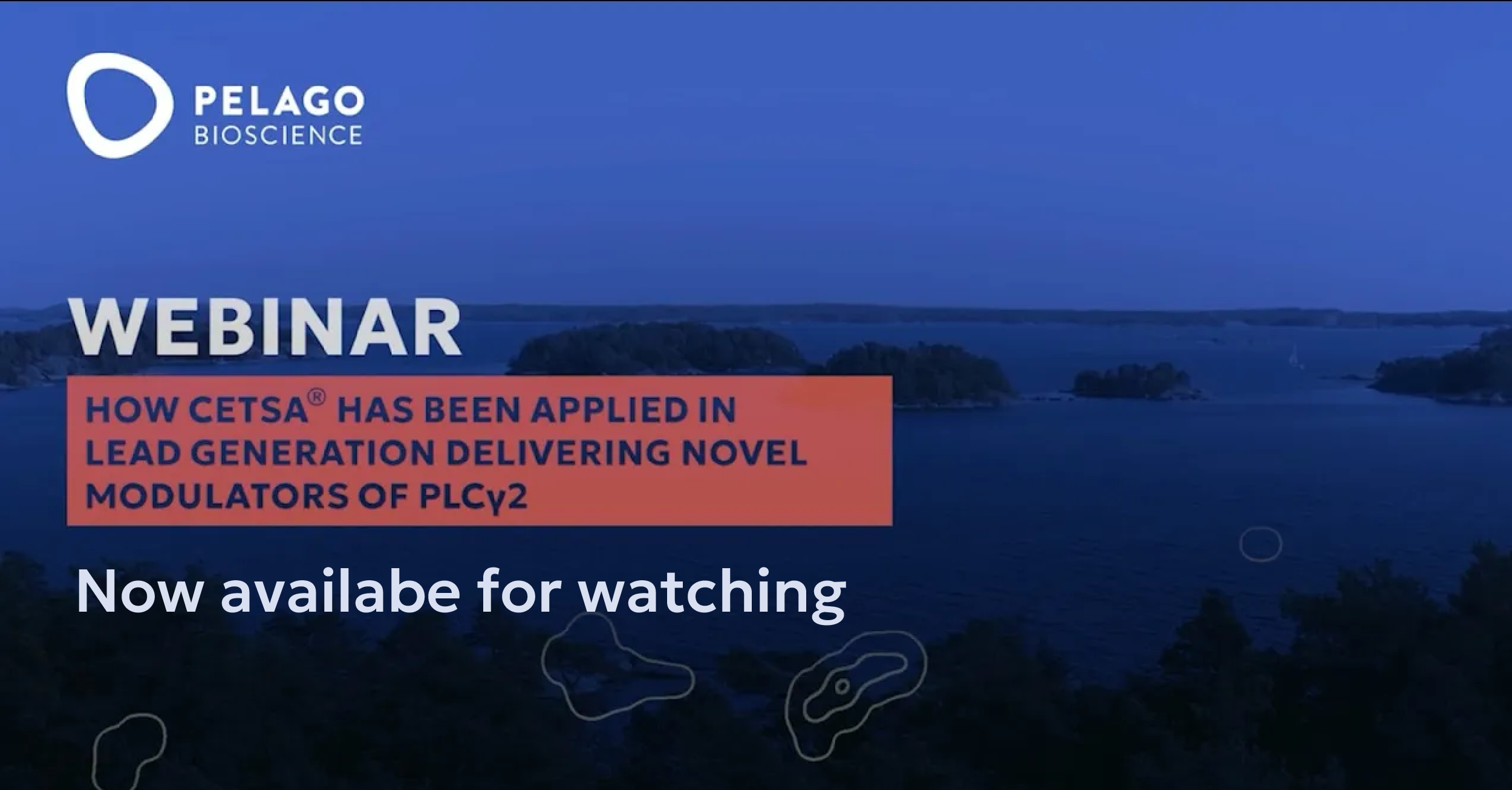
Uncovering Novel Modulators of PLCγ2 in Alzheimer’s Research with CETSA®
We are pleased to present our on-demand webinar, “How CETSA® has been applied in Lead Gen…